Summary
I became interested in structural biology as an undergraduate at the University of Oxford. This led me to start a PhD in 1999 with Venki Ramakrishnan at the MRC Laboratory of Molecular Biology in Cambridge. I worked as part of the team that determined the X-ray crystal structure of the small (30S) ribosomal subunit. In addition I solved structures of the ribosome bound to antibiotics and the protein initiation factor IF1. After my PhD I spent an extra year in Cambridge as a junior research fellow at Clare College, before moving in 2003 to Ron Vale’s lab at the University of California where I started working on dynein. Together with Sam Reck Peterson I used S.cerevisiae to express a recombinant dynein motor for biophysical studies. I collaborated with the group of Ian Gibbons to solve the structure of dynein’s microtubule binding domain (2008) and together with Carol Cho produced the first crystal structure of the dynein motor domain (2010). In 2008 I accepted a group leader position back at the MRC-LMB which I started in August 2010. My group intially focused on crystallography of the dynein motor domain, solving high resolution structures which revealed how ATP hydrolysis drives movement. We subsequently moved to asking how dynein works together with its cofactor dynactin to transport cargos, taking advantage of advances in cryoEM technology.
Longer Biographical Sketch
PhD at MRC LMB
I studied Biochemistry at the University of Oxford during a period that one of my professors described as a “golden age of structural biology”. The first crystal structures of myosin, the F1-ATPase, the nucleosome, nitric oxide synthase and the KcsA potassium channel were solved and made their way into our lectures. I was fascinated by the beauty of these structures, the way they would suddenly make sense of decades’ worth of data and the insight they gave into biology.
I therefore decided to apply for PhD positions in X-ray crystallography and was offered a chance to work on the 30S ribosomal subunit by Venki Ramakrishnan who was then at the University of Utah, but planning to move the MRC Laboratory of Molecular Biology in Cambridge. At that time no structure as large and asymmetric as the ribosome had ever been determined and my friends thought I was crazy to want to work on it. In the end, however, the excitement of the challenge and Venki’s assurances that he thought it would be possible made me sign on. It turned out to be a fortunate decision, because by the time I started in Cambridge, in the summer of 1999, Venki’s group had already made considerable progress, obtaining both crystals and a low resolution map of the structure. The task facing our team of six became one of trying to grow better diffracting crystals and solving the structure to a high enough resolution to be able to model all of the RNA and proteins.
My initial problem was that I struggled to grow crystals that were anything like as good as those prepared by others in the lab. In desperation I tried adding a cocktail of antibiotics that were known to bind the 30S ribosomes following a suggestion that adding ligands might aid the crystallisation process. Not only did it help me grow crystals but it also led to a structure of the 30S in the presence of three antibiotics (streptomycin, paromomycin and spectinomycin) which gave some fascinating insights into ribosome function and was eventually published alongside the structure of the 30S subunit.
Postdoc at UCSF
In 2003 I joined Ron Vale’s lab at the University of California San Francisco (UCSF). I had first met Ron when I was a still an undergraduate at Oxford. I was writing a final year paper on kinesin at the time and went along to a Royal Society meeting on motor proteins in London to try and get the latest scoop. Ron was very generous with his time and explained his lab’s latest data to me.
At Ron’s suggestion I started working on dynein. Initially I attempted to crystallize the 2MDa axonemal outer arm dynein complex purified from Tetrahymena. After about 200 litres of Tetrahymena culture it became clear to me that I would be better off working with a recombinant expression system. In particular, I wanted to be able to manipulate the dynein gene in order to test various different constructs in crystal trials. Samara Reck-Peterson had developed tools for expressing cytoplasmic dynein from S.cerevisae using the genomic copy of the gene. The beauty of this approach was that the dynein gene in yeast is non-essential and can be manipulated by homologous recombination. This made dealing with the 12kb dynein gene considerably easier than trying to use restriction enzymes to clone it into a plasmid. Sam taught me how to work with yeast and I set about using her system to overexpress different truncated versions of the dynein motor domain.
The study of the mechanism of kinesins and myosins was helped by having a dimeric version of the protein that moves processively (walks for multiple steps along the microtubule or actin filament without letting go). As a side project I decided to artificially dimerize the minimal dynein motor domains by fusing them to the constitutive dimer glutathione S-transferase (GST). As I had hoped these constructs were indeed processive and opened up the study of dynein to protein engineering, single molecule techniques and optical trap work. They were published in a collaborative study between myself, Sam Reck-Peterson and Ahmet Yildiz.
During my time in Ron’s lab I collaborated with Ian Gibbons, the discover of dynein, who had just come back from Hawaii and set up a lab as an emeritus scientist at UC Berkeley. Dynein binds to microtubules by a small domain at the tip of an antiparallel coiled coil. Ian was trying to express this microtubule binding domain (MTBD) for X-ray crystallography. To make stable constructs he fused it to a small stable protein (seryl-tRNA synthetase: SRS) that contained an internal antiparallel coiled coil. As he tested different fusion sites he noticed a pattern that some constructs bound to MTs well and others weakly. I realised the pattern could be explained if the two helices in the stalk coiled coil could slip past each other by about one turn of alpha helix. At the time this seemed like an outlandish prediction as coiled coils are generally thought of as very stable structures. It fitted, however, with some analysis I had done of the hydrophobic residues in the dynein stalk, which suggested that one of the two helices could easily adopt two different positions with respect to the other. Ian and I submitted our paper and to our utter surprise the reviewers recommended it was accepted for publication without any changes required. This is the only time in my career this has happened and turned out to be slightly problematic. I had spotted a small error in a supplementary figure and assumed there would be plenty of time to fix it. However, it was too late as the paper was already online. Despite Ian and my scepticism of our own model we were actually correct as was shown a number of years later.
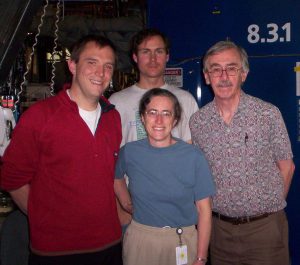
I continued my collaboration with Ian’s lab, helping his technician Joan Garbarino to crystallize one of his SRS-MTBD fusion constructs. We had crystals for several years, which looked beautiful but sadly diffracted to worse than 3.5Å. At this resolution my attempts to phase the structure were not successful and all our attempts to improve the crystals failed. Our breakthrough occurred when Ian hired a second technician called Wes Shippley. Joan and I showed him how to freeze crystals for data collection, explaining “that they wouldn’t diffract very well but at least the process would be good training”. To our amazement the crystals Wes froze diffracted to 2.2Å. Even better a round of molecular replacement, using the known SRS structure, followed by a round of refinement produced a beautiful map of dynein’s MTBD.
In the end we worked out what Wes had done. Protein crystals are less than a tenth of a millimetre across and grow in a small drop of liquid on a glass slide. To freeze them you flip the slide over, dip in a pin with a tiny thread loop on the end and then try and encourage the crystal to sit in the loop via surface tension. This is done while trying to watch what is happening through a microscope. Once the crystal is in the loop you pull the pin out of the drop of liquid and plunge it into liquid nitrogen. Wes, as with all first timers, was not sure whether he had got the crystal in the loop and so after pulling each crystal out of the drop had spent several minutes trying to see it under the microscope before freezing. This time-delay led to dehydration of the drop which caused the proteins in the crystal to pack more closely together and improved the diffraction. We had fun reproducing Wes’s result by fishing out a crystal, wandering round the Berkeley synchrotron waving it in the air for 2 minutes and then freezing it.
The MTBD structure was quite small but very beautiful and was the first part of the dynein motor to be solved. The contact site with the microtubule was linked directly to the coiled coil stalk suggesting how sliding of the helices could influence microtubule affinity. By the time our work was published in 2008 I had been lucky enough to be offered a group leader position back at the MRC-LMB. I decided to put the position on hold for two years in order to have one more go at what had been my first project: crystallizing the rest of the dynein motor domain.
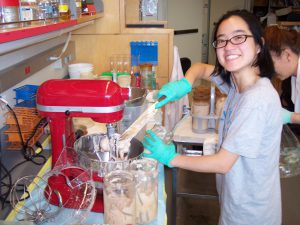
I was joined in this effort by Carol Cho, a PhD student who had previously done a rotation project with me. She had published her own paper and like me wanted to have another go at cracking dynein. We were joined by Nan Zhan: the lab manager, and Lan Jin: a technician we hired who had just finished her undergraduate studies. The yeast expression system produced nice protein but not enough for large-scale crystallisation trials. I therefore learnt how to grow more yeast, using a method called Fed batch fermentation: an approach used by the brewing and baking industry that allows one to grow up to 600 g of yeast per litre of culture. We used a 70 litre fermenter to produce over 14 kg each run. This produced so much yeast that we had to ask Ron to buy a new -80 freezer to store it in. Using a kilogram or two of yeast at a time we could produce sufficient protein for crystallisation trials and soon got our first hit. Using a method called seeding from that first hit we were able to produce lots of beautiful looking crystals. Their resolution was not great, around 5Å so Carol and I spent much of the next two years trying to improve the resolution and occasionally saw a crystal that diffracted to better than 4Å. We also spent many nights at the synchrotron upon the hill above Berkeley screening for heavy-metal derivatives that we could use to phase the structure. This was an exciting time because there were so many things to try. The process of screening was sometimes disheartening where crystal after crystal either showed either no-binding or no-diffraction.
Using a trick learnt from my ribosome days Carol and I soaked our crystals with a heavy metal cluster tantalum bromide. The diffraction was around 6Å, but there was a lot of phasing signal and the resulting maps were absolutely beautiful. To our delight they were quite easily interpretable because the motor domain is so rich in alpha helices which showed up in the map like tubes of density. I built in six AAA domains, and saw for the first time how dynein’s linker arched over the ring they made. Also exciting was seeing an unanticipated structure at the base of dynein’s talk. This came out of a neighbouring AAA+ domain and supported the stalk. It reminded me of the structure seen on British cathedrals called a buttress and so that is what I named it. By the time the structure was built it was almost time for me to return to the UK to start my own laboratory. As a way of celebration our small dynein group had several dinners together including a memorable visit to a Chinese restaurant at which Nan insisted we all ate chicken’s feet. And so, in the summer of 2010 I return to the UK and set up my lab. Over the next six months Carol and I also wrote up the paper. We presented the structure for the first time to the community at the ASCB meeting in Baltimore in December. We had a 15 minute presentation and did a double act of 7.5 minutes each.
To be continued…