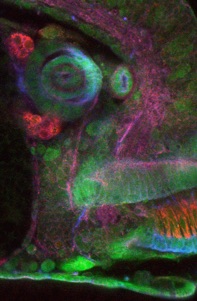
One of the most remarkable aspects of development is the formation of different tissues from seemingly very similar groups of cells. Most tissues derive from sheets of epithelial cells that deform and change shape, with each individual cell’s shape changes contributing to the overall change in tissue shape. For many processes such as tubulogenesis the transcriptional regulation that determines tissue identity has been uncovered, but the molecular basis of the complex shape changes that drive morphogenesis is not well understood. It will be important to decipher the regulation of morphogenesis in a healthy animal in order to be able to identify the underlying causes of disease phenotypes that emerge through misregulation of tubulogenesis, such as failure in tubule formation in polycystic kidney disease or uncontrolled proliferation without accompanying differentiation in cancers derived from epithelial tubular systems such as in breast cancer.
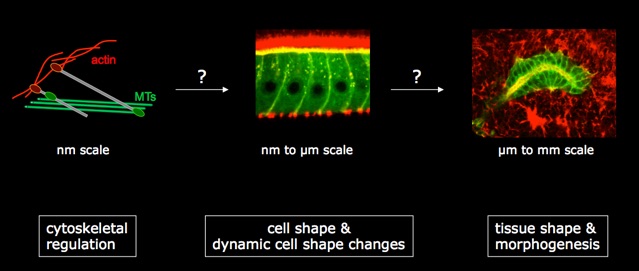
Cell shape is determined by the cellular cytoskeleton. Each cytoskeletal network is tightly controlled in its ability to expand and retract, but the different networks also need to be coordinated with each other, with cellular junctions and with neighbouring cells to form a final cellular shape appropriate for a certain tissue. Using Drosophila as a model organism, we are looking for proteins that regulate the cytoskeleton and cell shape using both genetic and candidate-based approaches. Excellent candidates for cytoskeletal regulators are the so-called ‘cytolinker’ proteins, proteins with the ability to interact with all cytoskeletal elements. We want to understand how a new family of cytolinkers, that we have recently shown to be essential for development in the fly, functions at a molecular level. Secondly, we have performed a genetic screen to identify novel proteins involved in cell shape regulation using the formation of the salivary glands as a model of tubulogenesis. We aim to characterize the candidate regulators identified in the screen to understand their molecular function and their impact on tissue morphogenesis. To understand how cell shape changes are integrated into the overall shape of a developing tissue, we are using live-imaging of salivary glands to follow groups of cells and analyse in detail how their shapes, positions and cytoskeletal components change through time.
Why use flies as a model system?
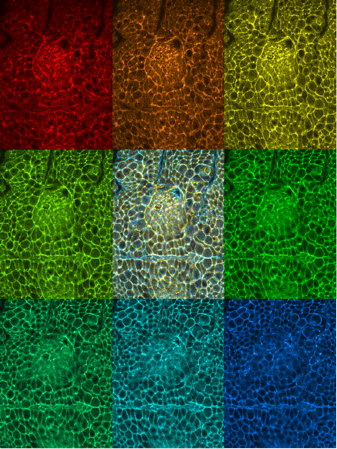
Drosophila offers many advantages to the developmental cell biologist:
The embryo develops outside the mother within 24 hours from a fertilized egg to a crawling larva with a functioning brain, muscles, digestive system etc. The life cycle of a fly takes about 12 days at room temperature, meaning that lots of flies can be grown in a short time (each female fly can lay up to several hundred eggs). The genome of Drosophila melanogaster and many related species has been sequenced, making forward and reverse genetic approaches very easy. Flies have been used as laboratory animals for more than a hundred years, ever since William Castle introduced them to the laboratory and Thomas Hunt Morgan and his group in New York performed their pioneering genetic work. Thus, an enormous variety of ever more sophisticated tools exist to generate mutation, knock-down protein levels, label proteins or even RNAs in vivo etc. Fly embryos and many adult tissues are completely translucent making them amenable for microscopic (fixed and live) analysis.
A new model: human renal organoids
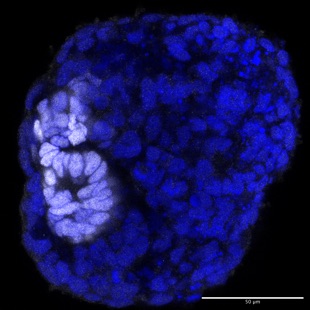
Many morphogenetic processes or modules that have been uncovered originally in simpler model organisms such as the fly or C.elegans have shown to be conserved throughout evolution into vertebrates and mammals.
The recent development of protocols to grow complex human tissue organoids from induced pluripotent stem cells in the lab has made the prospect of studying morphogenesis in a human model feasible.
We have recently established human renal organoids as a second model system in the lab to study firstly how the first epithelial polarity of the nephron is established during formation of the renal vesicle, a from of MET. Secondly, we want to understand the overall process of nephron tubulogenesis, the proliferation and expansion as well as segmentation of the nephron tube.