Timeline of contributions. Please note that this is not meant to be exhaustive or complete.
Hopefully it gives an idea of what we are doing or trying to do.
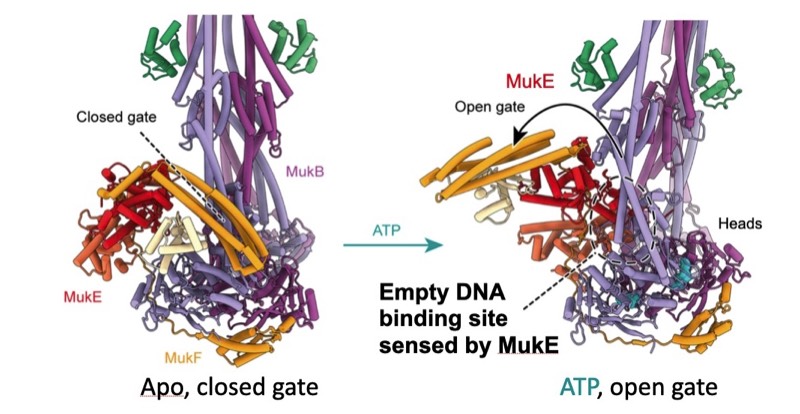
2025: Structure reveals pathway that loads DNA into the MukBEF SMC ring for topological entrapment. Ring-like structural maintenance of chromosomes (SMC) complexes are crucial for genome organisation and operate through mechanisms of DNA entrapment and loop extrusion. We explored the DNA loading process of the bacterial SMC complex MukBEF. Using electron
cryomicroscopy (cryo-EM), we demonstrated that ATP binding opens one of MukBEF's three potential DNA entry gates, exposing a DNA capture site that positions DNA at the open neck gate. We discovered that the gp5.9 protein of bacteriophage T7 blocks this capture site by DNA mimicry, thereby preventing DNA loading and inactivating MukBEF. We propose a comprehensive and unidirectional loading mechanism in which DNA is first captured at the complex's periphery and then ingested through the DNA entry gate, powered by a single cycle of ATP hydrolysis. These findings illuminate a fundamental aspect of how ubiquitous DNA organisers are primed for genome maintenance and demonstrate how this process can be disrupted by viruses. It also indicates that likely all SMC complexes entrap DNA topologically and that during loop extrusion DNA is topologically entrapped.
cryomicroscopy (cryo-EM), we demonstrated that ATP binding opens one of MukBEF's three potential DNA entry gates, exposing a DNA capture site that positions DNA at the open neck gate. We discovered that the gp5.9 protein of bacteriophage T7 blocks this capture site by DNA mimicry, thereby preventing DNA loading and inactivating MukBEF. We propose a comprehensive and unidirectional loading mechanism in which DNA is first captured at the complex's periphery and then ingested through the DNA entry gate, powered by a single cycle of ATP hydrolysis. These findings illuminate a fundamental aspect of how ubiquitous DNA organisers are primed for genome maintenance and demonstrate how this process can be disrupted by viruses. It also indicates that likely all SMC complexes entrap DNA topologically and that during loop extrusion DNA is topologically entrapped.
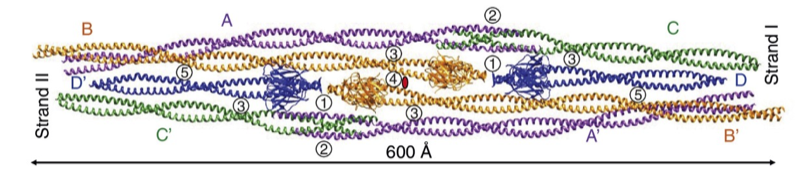
2024: Structure of bacterial intermediate filament protein crescentin. Crescentin is a coiled coil protein that is required for the crescent cell shape of bacteria such as Caulobacter crescentus. Crescentin shares biochemical and cytoskeletal properties with intermediate filament (IF) proteins, which form the third major class of cytoskeletal proteins in eukaryotes. To better understand the relationship between crescentin and IF proteins, and the filaments they form, we have determined the three-dimensional structure of crescentin filaments by cryo-EM. This revealed the full-length structure of the parallel coiled coil dimer of crescentin and how dimers come together laterally and longitudinally, to form a non-polar, octameric filament. Differences in filament architecture highlight the versatility of intermediate filament-like proteins across the tree of life.
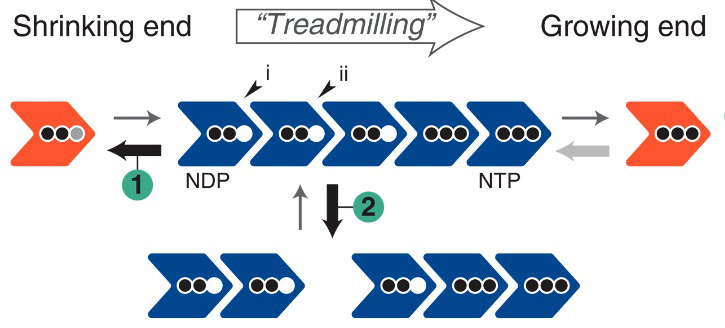
2023: Cytomotive switch is required for actin and tubulin filaments to do useful dynamics. Protein filaments are used in myriads of ways to organize other molecules within cells. Some filament-forming proteins couple the hydrolysis of nucleotides to their polymerization cycle, thus powering the movement of other molecules. These filaments are termed cytomotive. Only members of the actin and tubulin protein superfamilies are known to form cytomotive filaments. We examined the basis of cytomotivity via structural studies of the polymerization cycles of actin and tubulin homologs from across the tree of life. We analyzed published data and performed structural experiments designed to disentangle functional components of these complex filament systems. Our analysis demonstrates the existence of shared subunit polymerization switches among both cytomotive actins and tubulins, i.e., the conformation of subunits switches upon assembly into filaments. These cytomotive switches can explain filament robustness, by enabling the coupling of kinetic and structural polarities required for cytomotive behaviors and by ensuring that single cytomotive filaments do not fall apart.
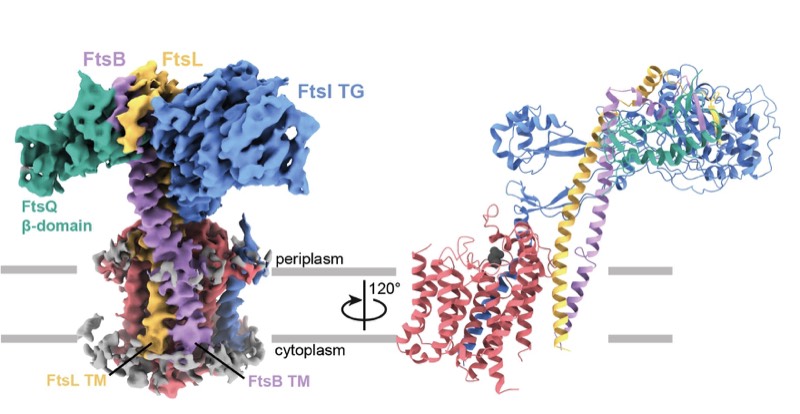
2023: Finally, divisome core complex resolved. Cell division in the vast majority of bacteria depends on the synthesis of new cell wall peptidoglycan and is catalysed by the divisome. Central to the divisome is the peptidoglycan-synthesising protein complex FtsWI, which is regulated by the heterotrimer FtsQBL. We have now resolved the cryo-EM structure of the activated divisome catalytic core complex FtsWIQBL from Pseudomonas aeruginosa at 3.7 Å resolution. The structure reveals details of the periplasmic interfaces within FtsWIQBL, including the positioning of FtsI by the coiled coil of FtsBL, as well as a transmembrane domain containing FtsWIBL, but not FtsQ. We are able to provide molecular mechanisms of a multitude of cell division phenotypes of known mutations and reveal a large conformational switch between presumably inactive and active states of the FtsWI core enzymes. This is the beginning of a longer journey into the molecular mechanism of FtsZ-based bacterial cell division.
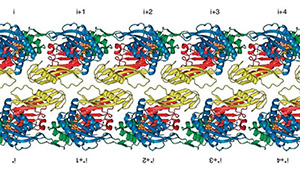
2022: FtsA antiparallel double filaments are curved, making it likely that actin-like FtsA functions as a rudder in cell division, as does MreB in cell elongation. During bacterial cell division, filaments of tubulin-like FtsZ form the Z-ring, which is the cytoplasmic scaffold for divisome assembly. In Escherichia coli, the actin homologue FtsA anchors the Z-ring to the membrane and recruits divisome components, including bitopic FtsN. FtsN regulates the periplasmic peptidoglycan synthase FtsWI. To characterize how FtsA regulates FtsN, we applied electron microscopy to show that E. coli FtsA forms antiparallel double filaments on lipid monolayers when bound to the cytoplasmic tail of FtsN. Using X-ray crystallography, we demonstrate that Vibrio maritimus FtsA crystallizes as an equivalent double filament. We identified an FtsA–FtsN interaction site in the IA–IC interdomain cleft of FtsA using X-ray crystallography and confirmed that FtsA forms double filaments in vivo by site-specific cysteine cross-linking. FtsA–FtsN double filaments reconstituted in or on liposomes prefer negative Gaussian curvature, like those of MreB, the actin-like protein of the elongasome. We propose that curved antiparallel FtsA double filaments together with treadmilling FtsZ filaments organize septal peptidoglycan synthesis in the division plane.
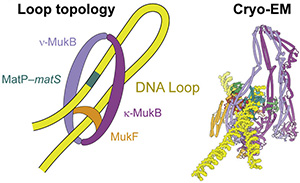
2021: Structure of MukBEF:MatP solved. We have solved the complete cryo-EM structure of the SMC complex of E. coli, MukBEF, bound to DNA and its DNA unloading factor, MatP. The arms of the DNA loop are threaded through separate compartments of the MukBEF complex, keeping the DNA loop ‘double locked’ to promote length-wise compaction of the chromosome. This is the first time two DNA strands have been resolved in SMC complexes. It indicates that a loop is topologically entrapped inside the complex, with the kleisin chain (MukF) going through the loop, preventing it from slipping out during loop extrusion. In order to test whether the mode of DNA binding revealed by cryo-EM also occurs in cells, we genetically engineered E. coli strains in which the SMC ring could be chemically locked. Chromosomal DNA was indeed trapped inside the ring in these modified E. coli cells, suggesting that MukBEF does enclose DNA loops in cells – as observed in the cryo-EM structure.
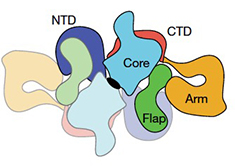
2020: Structure of human thyroglobulin solved. Thyroglobulin (TG) is the protein precursor of thyroid hormones, which are essential for growth, development and the control of metabolism in vertebrates. Hormone synthesis from TG occurs in the thyroid gland via the iodination and coupling of pairs of tyrosines, and is completed by TG proteolysis3. Tyrosine proximity within TG is thought to enable the coupling reaction but hormonogenic tyrosines have not been clearly identified, and the lack of a three-dimensional structure of TG has prevented mechanistic understanding. We solved the structure of full-length human thyroglobulin at a resolution of approximately 3.5 Å, determined by cryo-electron microscopy. We identified all of the hormonogenic tyrosine pairs in the structure, and verified them using site-directed mutagenesis and in vitro hormone-production assays using human TG expressed in HEK293T cells. Our analysis revealed that the proximity, flexibility and solvent exposure of the tyrosines are the key characteristics of hormonogenic sites. We transferred the reaction sites from TG to an engineered tyrosine donor–acceptor pair in the unrelated bacterial maltose-binding protein (MBP), which yielded hormone production with an efficiency comparable to that of TG. Our study provides a framework to further understand the production and regulation of thyroid hormones. An important structure, justifying a diversion from our usual trajectory!
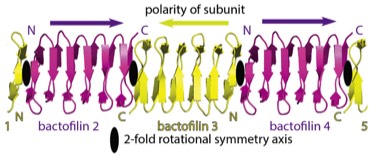
2019: Structure of bactofilin filaments. Actin and tubulins of prokaryotic cytoskeletons get the most attention but it is a fact that other filament systems are also very widely distributed across archaea and/or bacteria. One such filament system is bactofilins. Recognised only in 2010 as cytoskeletal proteins, bactofilins are small beta helical proteins that form constitutive, non-dynamic filaments close to membranes. Through the use of modern high-resolution cryo-EM and crystallography, we have determined the structure of a bactofilin filament from Thermus thermophilus and determined that they form non-polar filaments by stacking the small domains into one continuous beta helix, explaining their amazing biochemical resistance to de-polymerisation. We also show that all bactofilins polymerise this way using co-evolutionary analysis and that bactofilin filaments bind to membranes directly through their N-terminal tails. Bactofilins are also present in some eukaryotic organisms such as oomycetes and although we show one of these to polymerise, it is anticipated that their presence in eukaryotes is because of horizontal gene transfer.
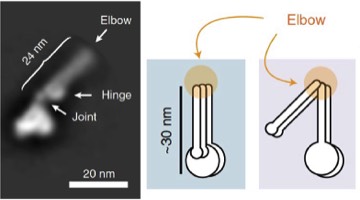
2019: A folded conformation of SMC complexes. Almost all cells contain protein complexes that have SMC proteins at their heart, such as eukaryotic cohesion, condensin and bacterial Smc/ScpAB and MukBEF complexes. Based on old EM data it has been assumed for a long time that the characteristically long coiled coil segments of the SMC proteins are structural, keeping the ATPase head domains and hinge domains apart. By using a combination of EM and cross linking methods we now show that most likely all these complexes fold their coiled coils at a site we now call the 'elbow', leading to a dramatically different shape of the molecules, and bringing heads and hinges close together. This conformational change is expected to play an important role in the DNA transactions the complexes facilitate, such as DNA translocation.
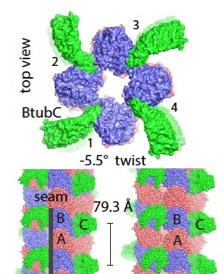
2017: Bacterial 'mini microtubules'. Most if not all eukaryotic microtubules have large protofilament numbers - they form hollow cylinders made out of 11-13 protofilaments, themselves consisting of alternating alpha and beta tubulin subunits. The bacterial tubulin homologue FtsZ, involved in cell division, does not form any such structures. We could now show that BtubA and BtubB protein from P. dejongeii form microtubules that are made out of 4 protofilaments. These 'mini microtubules' share many properties with eukaryotic microtubules, just not the protofilament number. They are hollow, contain alternating A/B protofilaments, are held together by M-loops, are dynamic and show dynamic instability, and the dynamics are regulated by another protein, called BtubC that makes the mini microtubules completely stable.
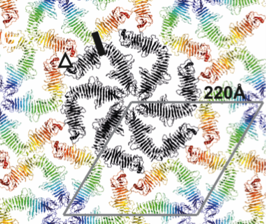
2017: In situ S-layer structure. Most archaea and many bacteria are coated with a single layer of protein - called a surface layer or S-layer. It is thought that S-layers protect cells from phages and large molecules, they give cell membranes stability and may also help the cells to acquire certain chemical properties. Using a combination of cellular electron cryo tomography (cryo-ET) with subtomogram averaging and X-ray crystallography we determined the structure of the complete and intact S-layer lattice on Gram negative Caulobacter crescentus cells. The combination of the two methods enabled us to show that the lattice formed in the crystals matches perfectly the lattice formed on cells as determined at 7.4 Å directly on cells. The S-layer-forming RsaA protein is almost completely a beta-helical fold and arranges into large hexamers to form the core of the lattice. Opening of maximal 27 Å are the result, excluding very effectively everything from very small peptides upwards. Calcium ions play important roles both for the stability of the RsaA monomer but also for the lattice, explaining its dependence on calcium ions.
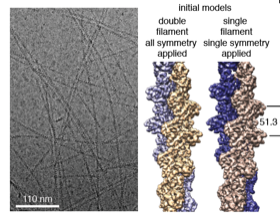
2016: Crenactin forms double-helical F-actin-like filaments. Crenactin is closer in sequence to eukaryotic F-actin than any other bacterial or archaeal protein. Here we show that crenactin forms double helical filaments that are very closely related to F-actin, the structure that all bona fide actins form in eukaryotes. Given the sequence similarity this might not be seen as so surprising but previous work claimed this was not the case and also the hydrophobic plug, a loop on the back of the fold, was seemingly in the way of filament formation when crystal structures of crenactin were previously determined. We also show that arcadin-2 effectively inhibits crenactin filament formation through binding to the hydrophobic cleft on crenactin, another parallel to eukaryotic F-actin, this time its regulation.
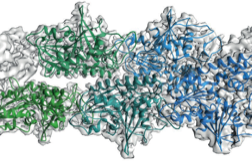
2015: ParM doublet structure and asynchronous mechanism. We have previously shown that actin-like ParM filaments push plasmids apart during their segregation through the formation of an antiparallel spindle. We have determined the structure of ParM from cryoEM data to 4.3 Å resolution, enabling standard atomic refinement procedures to be employed to obtain a reliable atomic model of the entire double-helical filament. Again using cryoEM we imaged the doublets that form when two double helical filaments come together to form the spindle and these where also imaged directly in E. coli cells by tomography. Since we only found doublets in cells and not bundles, an asynchronous mechanism of ParMRC-dependent plasmid segregation was proposed, obviating the need for cohesion and spindle checkpoints.
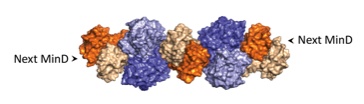
2014: MinCD filaments discovered. We have discovered that the MinC and MinD proteins of the septum-site selection system MinCDE form cytomotive filaments. Through MinD, the filaments are anchored to the cell’s membrane and consist of alternating dimers of MinC and MinD. MinD’s ATPase activity makes the filaments cytomotive and it is expected that the filaments activate MinC to be able to interfere with FtsZ polymerisation, although the exact mechanism remains to be elucidated.
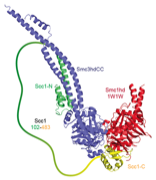
2014: Cohesin ring closed. Cohesin is thought to form a giant tripartite ring around sister chromatids during eukaryotic mitosis, consisting of the proteins Smc1, Smc3 and Scc1. We have revealed the last unknown interface forming the ring, formed by the N-terminal domain of Scc1 and the ATPase head domain of Smc3. Unexpectedly, the Scc1 domain binds to the coiled coil region of the SMC head, making this interaction completely different to the Scc1:Smc1 interaction. Having visualised all three interfaces makes the ring model of cohesion action much more robust.
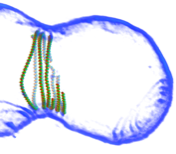
2014: FtsZ ring constriction reconstituted in liposomes. Adding FtsZ and FtsA to the inside of small liposomes leads to spontaneous constriction! This we then imaged with high-resolution electron cryotomography, producing pseudo-atomic pictures (as the one on the left) of an constricting FtsZ ring. These data lead us to a mechanism involving sliding filaments within the Z-ring structure.
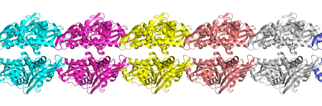
2014: Structure of MreB filament revealed. Using in vivo crosslinking, electron microscopy and crystallography, we have revealed the filaments MreB forms in cells. Surprisingly, MreB filaments are straight, antiparallel and have no polarity, in contrast to actin and ParM. High-resolution crystal structures of Caulobacter MreB also revealed the binding mode of the MreB inhibitor A22 and conformational changes that accompany filament formation since we obtained crystals in monomeric and double-filament states.

2014: Crystal and filament structure of crenactin. This protein was discovered by Rolf Bernander (Uppsala, Sweden) and the structure shows it to be closely related to actin, much more so than 'normal' prokaryotic actin MreB. Since crenactin, as its name suggests occurs in crenarchaea, this might mean that crenarchaea have something to do with the formation of eukaryotes; crenactin might be the closest so far we have seen to a precursor of all eukaryotic actins.
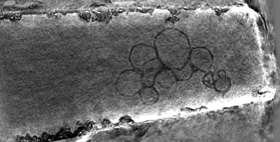
2013: Cylindrical specimen holder for electron cryotomography. In order to avoid the 'missing wedge' problem in electron cryotomography, leading to severe anisotropy, we developed carbon micropipettes that produce the same thickness at any tilt angle from 0 to 180°.

2012: Bipolar spindle formation by ParMRC through filament bundling. The ends of dynamically unstable ParM filaments come together in an antiparallel way, both protecting the minus (pointed) ends from disassembly and enabling the formation of a bipolar spindle, segregating all sister plasmids at the same time.
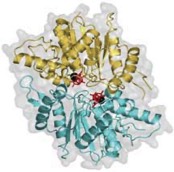
2012: MipZ dimer crystal structure and mechanism with Martin Thanbichler (MPI Marburg). MipZ belongs to the MinD deviant Walker A family of small ATPases.
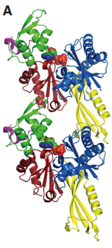
2012: FtsA actin-like protofilament formation demonstrated. In 2000 we showed that FtsA has an unusual actin-like fold. We demonstrated here that FtsA is actually a domain swap, still enabling the formation of canonical actin-like protofilaments. This was supported by structures and in vivo light and electron microscopy of filaments.
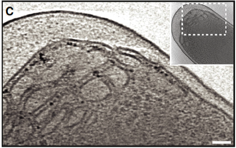
2011: Developed a ferritin label for electron cryotomography of cells. Using the electron dense core of 24-meric ferritin, expressed in E. coli, we visualised the label and showed that its position in the cell faithfully resembles locations of known proteins it was fused to. This represents our first attempt at a GFP-analogue for electron cryotomography. The label is currently multivalent and we are working on improving this. It needs to be monovalent to be useful.
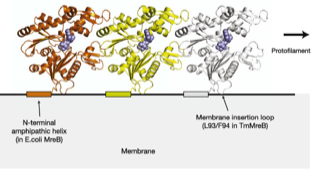
2011: Direct membrane attachment of MreB discovered. We discovered that all MreBs contain sequences that bind to membrane directly. Gram negative MreBs do this via an N-terminal amphipathic helix. This explains how MreB is oriented in cells, why MreB so far has been invisible in electron cryotomograms and why the proteins are generally difficult in vitro.
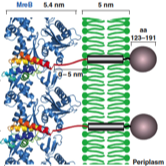
2010: Structure of MreB in complex with RodZ. First co-crystal structure of MreB.
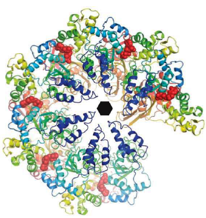
2010: Crystal structure of Lon protease. Our attempt at solving the last missing crystal structure of the large size-exclusion proteases, Lon.
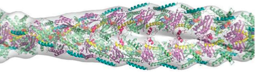
2010: Crystal structure of TubZ. TubZRC is a tubulin-based plasmid partitioning system discovered earlier by Joe Pogliano (UC San Diego). Here we show that TubZ is indeed related to tubulin in fold, but forms double-helical filaments that are somewhat reminiscent of actin! It highlighted to us that cytomotive filament systems always conserve the fold and protofilament, but are quite flexible when it comes to the actual super-structure of the filament, probably tailored to the task required by their functions.
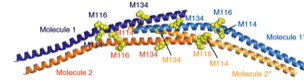
2010: DivIVA crystal structure and membrane attachment mechanism. DivIVA replaces MinE in some Gram positive organisms and here we presented the composite structure of this amazing protein. It does look a bit like membrane-bending eukaryotic Bar domains and it indeed binds to membrane as demonstrated biochemically.
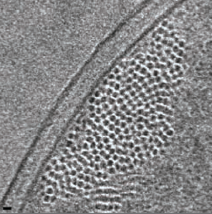
2009: Cryosections of E. coli cells show bundles of ParM filaments. By using different expression levels of ParM in E. coli cells, we demonstrated that the filaments can be visualised in cryo sections of these cells without any labelling and without any doubt. By moving to the wild type levels, we showed that 3-7 filaments form bundles in cells segregating plasmids. This number is close to the copy number of the R1 plasmid, which is around 5. This suggested that the plasmids are segregated together in a bundle of sisters, by a bundle of filaments.
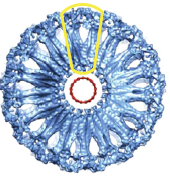
2009: EM reconstruction of bacterial dynamin lipid tubes. Bacterial dynamin from Nostoc forms ordered lipid tubes very efficiently in vitro, bending the lipid membrane into a very small diameter. A semi-atomic model of the resulting structure showed how the bending occurs and how the protein assembles in order to facilitate bending of the lipid membrane.
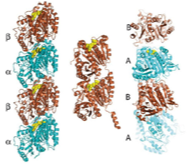
2008: Term ‘cytomotive’ suggested. We believe that the term cytoskeleton is misleading. In bacteria (and also to some extent in eukaryotes), the filaments formed by actin- and tubulin-like proteins function through polymerisation and de-polymerisation and are not used as a skeleton in the mechanical sense. It seems to us that the original function of these proteins was as one-dimensional motors, generating force through assembly and disassembly. To distinguish these from other, static filament systems we invented the term ‘cytomotive filaments’.
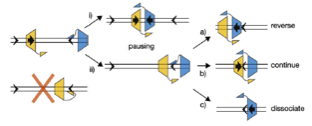
2008: Understanding of the mechanism of directionality of FtsK with Dave Sherratt (Oxford). The FtsK DNA translocase uses special KOPS loading sequences that tell it which direction to go. We were able to show that this is facilitated by the C-terminal gamma domain of FtsK that loads in a sequence specific way and thus orients the motor before it starts moving. A crystal structure of the gamma domain bound to KOPS was presented.
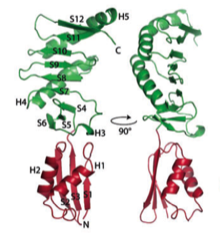
2008: Structure of FtsQ. After a long struggle with the crystals, we finally solved the crystal structure of FtsQ, a protein that is central to the divisome, the protein complex that forms with the FtsZ ring during bacterial cell division.
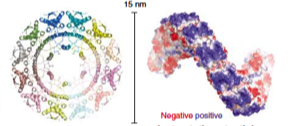
2007: Crystal structure of ParR. ParR belongs to the ParMRC plasmid partitioning system. The crystal structure showed an unexpected superhelical arrangement, with DNA binding on the outside, wrapping around the helix. This way, ParR binds to an extended stretch of the parC centromeric DNA on the plasmid.
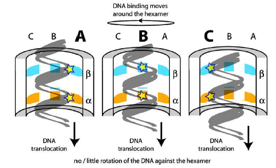
2006: Crystal structure of the hexamer of FtsK with Dave Sherratt (Oxford). FtsK is a DNA translocase, moving at around 5 KBp/s along double-stranded DNA. We showed that FtsK forms a hexamer, with the DNA going through the middle of the ring.
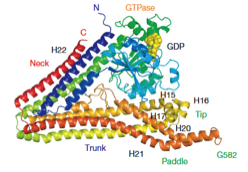
2006: Discovery and structure of bacterial dynamin. Several bacteria contain genes that are related to eukaryotic dynamins. Lipid tubulation is described as well as the crystal structure of these lipid-bending proteins. The biological function of these proteins is still unclear today (2012).
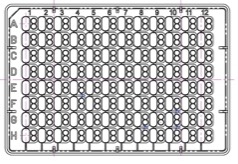
2005: Original MRC crystallisation plate brought to market. After being frustrated with all available plates at the time, we designed our own crystallisation plate for robotic drop setting in SBS and 96 well format in collaboration with Paul Reardon at SWISSCI. The plate is made and distributed by SWISSCI and we have now a whole family of MRC plates available for different tasks.
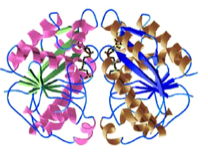
2005: Structure of Soj dimer, a protein of the MinD family. First crystal structure of a ‘MinD’ –like dimer. The family of deviant Walker A proteins are predicted to all form dimers and we showed here why the P-loop has to have the special signature motif. A mutant is described that helps with more stable dimer formation. First description of DNA-binding activity by Soj.
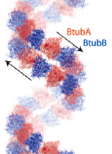
2005: Structure of BtubAB, a horizontally transferred bacterial tubulin. A rare glimpse of horizontal gene transfer. BtubAB is closely related to tubulin (50 % identity in sequence) and the structures are remarkably similar. Yet, BtubAB does not need any unusual co-factors for folding in E. coli. We believe this is sign of horizontal gene transfer because the parent organism, Prosthecobacter dejongeii contains no other genes that are so unusually closely related to eukaryotic proteins.
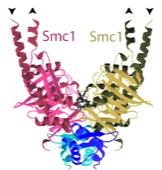
2004: SMC1:kleisin interaction structure with Kim Nasmyth (Oxford). First view of the interaction of the Scc1 kleisin C-terminal winged helix domain and the SMC1 head domain. This is one half of the cohesin ring closure. The other half is still missing today (2012).
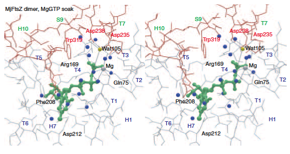
2004: Crystal structure of FtsZ protofilament interaction. Finally, a crystal structure of the FtsZ protofilament interaction. Resolution was good enough to see the chemistry of water attack of the gamma phosphate of the nucleotide. The dimer shown is bent and probably represents a pre-filament state that will only form after hydrolysis, when the FtsZ subunits move closer.
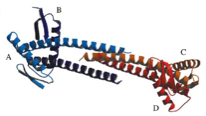
2004: Crystal structure of FtsZ-interacting protein ZapA. Structure of one of the many FtsZ-interacting proteins. It forms a dimer or tetramer and it is still not clear today (2012) what this protein does to FtsZ and how.
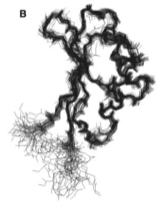
2004: NMR structure of FtsN. FtsN is a late cell division protein and we showed that only the very C-terminal part of the protein is folded in isolation. The middle section, after the membrane anchor, is unfolded, possibly providing a spacer to the cell wall through periplasm.
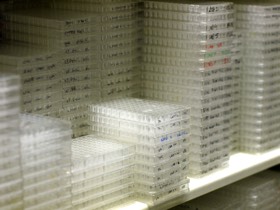
2003: Full operation of the Crystallisation Facility. The facility, started with Daniela Stock (Neurobiology Division) and Olga Perisic (PNAC Division), finally became fully operational in 2003 and started to serve the whole LMB. We automated screen reformatting, screening and optimisation.
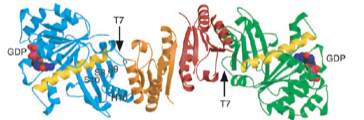
2003: Crystal structure of SulA in complex with FtsZ. SulA was shown to block the protofilament of FtsZ, immediately explaining the sequestration mechanism of SulA’s action on cell division during the SOS stress response, through inhibition of FtsZ.
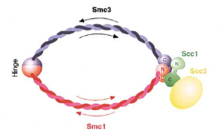
2002: Ring model of cohesin published with Kim Nasmyth (then IMP Vienna, now Oxford). Through the structure of the SMC hinge domain it became clear that SMC proteins form intermolecular dimers. This enabled interpretation of the interaction data of the kleisin and the SMC and led to the ring model of cohesin (and possibly all SMC-protein containing complexes).
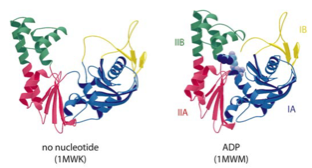
2002: Structure of the plasmid segregation protein ParM. ParMRC is a plasmid partitioning system of the R1 plasmid of E. coli. Here we showed in collaboration with Kenn Gerdes (then Odense, Denmark, now Newcastle) that ParM is indeed related to actin in structure and that it forms double helical filaments, as demonstrated by electron microscopy and image reconstruction.
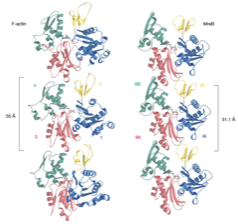
2001: Prokaryotic ancestor of actin discovered. Just after Jeff Errington's landmark paper on MreB (then Oxford, now Newcastle), we described MreB's structure and the protofilament it forms. It turns out that the structure is remarkably similar to actin, despite only 20 % sequence identity. Furthermore, the protofilament is very similar, too, relating to one strand of the double helical F-actin filament.
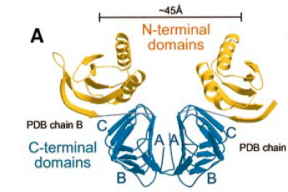
2001: Crystal structures of the MinC and MinD cell division inhibitors. The MinCDE cell division inhibitor is involved in the correct positioning of FtsZ during bacterial cell division. The MinC crystal structure showed an unexpected dimer formed by a beta helix domain.
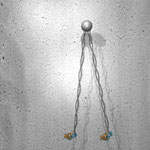
2001: SMC protein head crystal structure. SMC proteins are V-shaped with ATPase domains at the two open ends. Here we showed that the ATPase domain belongs to the ABC fold and that the arms of the V are formed by very long insertions into the canonical ABC ATPase fold.
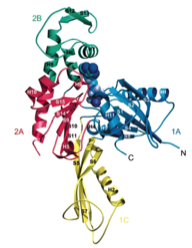
2000: Crystal structure of FtsA. FtsA is a partner of FtsZ in bacterial cell division and here we showed that FtsA is a derivative of the actin-fold, with one subdomain removed and a different one, 1C, added. This raised doubts whether FtsA would actually be able to polymerise. We did show this finally in 2012.
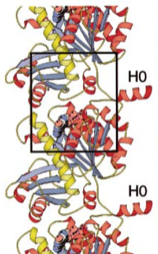
1999: Tubulin-like protofilaments of FtsZ. Through the use of a 3D electron microscopy reconstruction we showed that FtsZ and tubulin also share the same filament architecture. This is important because nucleotide hydrolysis is linked to polymerisation in these proteins.
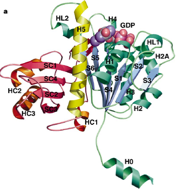
1998: Crystal structure of FtsZ from Methanococcus jannaschii. Direct proof was obtained that the FtsZ protein, which is involved in bacterial cell division (cytokinesis) shares the same fold with eukaryotic tubulin, despite having less than 20 % sequence identity.