Identification of sequence whereby SARS-CoV-2 Spike protein bypasses internal protein packing organelle driving syncytia formation to further spread infection
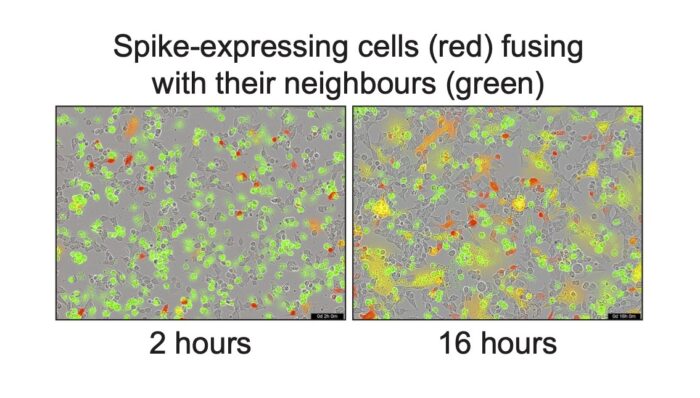
The Spike protein of SARS-CoV-2 protrudes out from the lipid membrane of the virus and binds to the membranes of cells in our lungs, thus allowing the viral contents to infect the cell where it can replicate and then spread to more cells. After infection, the virus programmes the cell to replicate the viral proteins, including the Spike protein. Most of these new Spike proteins accumulate in an intracellular structure named Golgi which assembles new viruses. However, some of the Spike proteins travel past the Golgi and reach the surface of the cell where they can bind to neighbouring cells. Just as Spike directs the fusion of the virus to a cell, at the surface it can cause the infected cell to fuse to its healthy neighbours, resulting in the formation of giant cells called syncytia, a characteristic of SARS-CoV-2 infected lungs. Syncytia formation allows the virus to spread between cells without ever needing to leave the cell, thereby avoiding exposure to the immune system. Now, Sean Munro’s group, assisted by Leo James’ group, have examined how these Spike proteins move through infected cells and found how the accumulation of Spike at the surface of the cell to produce syncytia is enabled.
Most of the Spike protein exists externally to the virus, but it also has a transmembrane domain and a short cytoplasmic tail which is inside the cell. In examining why Spike acts in the manner it does, Sean’s group found that several proteins of the invaded cell bind to Spike’s short tail and direct it to the Golgi apparatus, then hold it there whilst new viruses are made. However, this mechanism isn’t as effective as the comparative one used by cellular proteins which stay in the Golgi, as some of the Spike proteins ‘leak’ through to the cell surface where they can direct fusion to neighbouring cells. Furthermore, the group found that once Spike reaches the cell surface, it does not recede back into the cell. This drew the group to the conclusion that the cytoplasmic tail of Spike is optimised to ensure that some of the Spike proteins escape the Golgi to make it to the surface where they can cause syncytia to form.
In testing this, Lawrence Welch and Jérôme Cattin-Ortolà made a copy of the Spike tail in bacteria, and used it to identify binding proteins from cell lysates. Here they found several proteins usually used by the cell for moving its own membrane proteins between intracellular structures and delivering them to the cell surface. In mapping which parts of the tail bind to each protein, they discovered that a recognition motif within the Spike protein which is responsible for binding with a Golgi-retaining complex, possessed a suboptimal histidine residue which encourages premature detachment. This facilitates some Spike proteins to bypass the Golgi to reach the cell surface.
These findings represent a greater biological understanding of the SARS-CoV-2 virus. In highlighting the importance of syncytia to the infection, the study also suggests that small molecules which block syncytia formation could prove useful in restricting the spread and detrimental effects of the virus. Furthermore, all of the vaccines currently in use rely on expressing the whole Spike protein from mRNA or adenovirus vectors, and typically contain small mutations which block its ability to form syncytia. Therefore, it might be possible to further improve the efficacy of these vaccines by altering the cytoplasmic tail of Spike to modulate the amount that appears on the surface of cells.
This work was funded by UKRI MRC.
Further references
Sequences in the cytoplasmic tail of SARS-CoV-2 Spike facilitate expression at the cell surface and syncytia formation. Cattin-Ortolà, J., Welch, L., Maslen S. L., Papa, G., James, L. C., Munro, S., Nature Communications 12, 5333 https://doi.org/10.1038/s41467-021-25589-1
Sean’s group page
Leo’s group page
LMB COVID-19 response