From the scientist’s view: a conversation with… William Schafer
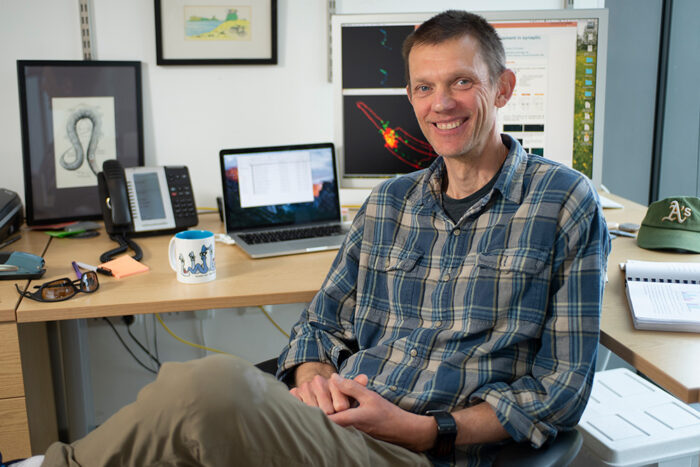
William Schafer is a Group Leader in the Neurobiology Division at the LMB.
William was born in the Unites States of America, and he studied his undergraduate degree at Harvard University. He then went on to study his PhD at the University of California, Berkeley, which focused on the lipid modification of proteins in the laboratory of Jasper Rine.
As a postdoc, William’s research changed to focus on C. elegans under the supervision of Cynthia Kenyon at the University of California, San Francisco (UCSF). His work focused on the connection between behaviour and the nervous system and signified William’s first formal introduction into Neurobiology research. William became an Assistant Professor at the University of California, San Diego (UCSD) where he worked for 10 years.
He first came to the LMB on a sabbatical year in 2004. His interest in continuing to work at the LMB was evident and, after returning to UCSD for a year, William formally moved his lab to the LMB in 2006.
William’s work has primarily focused on the nervous system of C. elegans, including exploring how sensory pathways work, how neuro modulators control behavioural states, and neuro peptides binding receptors. Most recently, his groups’ research has expanded beyond C. elegans to other animals as well.
We recently spoke to William about his research and career in science. Here are some of the interview highlights, or scroll to the bottom of the page to find the full video.
What inspired you to choose this particular area of research?
When I was a graduate student I worked on yeast, within cell biology, but I also really enjoyed the process of using genetics to try to understand problems. At the time I was not somebody who was particularly handy at the bench, but I could always figure out how to do a genetic cross and try to interpret it. I was interested in the brain, and I thought it would be interesting to try to study how the brain worked. The best way that I could approach that problem was through using genetics. I thought if I want to use genetics to study the brain, I could either work in C. elegans or I could work on flies.
At the time, Cynthia Kenyon didn’t really work on neuroscience. She worked on developmental biology, but she was interested in having me work in her lab on a neuroscience project. She was very supportive, and it was great.
What are the advantages of using C. elegans nematode worms?
The nematode was chosen as an experimental organism because you can do molecular genetics and crosses at the bench, like you are working with bacteria or yeast. You set up crosses, make mutants, and try to figure out what genes are defective in the mutant, and then you study its phenotype.
One of the things that I have been interested in from the beginning, is trying to use automated machine vision microscopy to try to quantify behavioural phenotypes that we see in worms. You can make a little automated microscope that will make long movies of worms over time. And then you can take these videos and try to extract features of the behaviour that are relevant to how the brain works. So, there’s lots of people that spend their time doing these worm tracking experiments as well.
The third thing that we were amongst the first to do is to use genetically encoded fluorescent probes to try to visualize neural activity while worms are doing behaviour. We can express modified fluorescent proteins that are sensitive to neural activity, and then we can look and see how particular identified neurons in circuits respond to a particular stimulus. So those are some of the techniques that we’ve used for nearly 30 years in relation to worms.
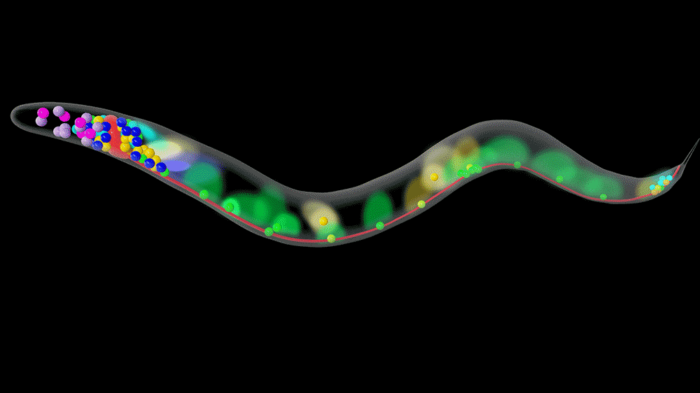
What other animal models are important to your research?
We have recently been doing more electrophysiological experiments. You take worm genes and produce their RNA and inject it into frog eggs. From these frog eggs, you can stick electrodes into the oocyte, and you can record the activity of the worm. Then you can use a two-electrode voltage clamp technique to record the activity of the channel or receptor from the worm in an exogenous environment. So, you can look in isolation at a particular channel or receptor, and we have automated two electrode voltage clamp rigs. This is the technique that was our way in to start studying the octopus. You can inject octopus genes and explore the properties of octopus neurotransmitter receptors. Once we found interesting features of these receptors, then we could go back to the octopus brain and see where they were in the brain.
Has there been an experiment or piece of work that didn’t go to plan, but turned out to have a positive, if unexpected, outcome?
One example I can think of was when a student was working on neural circuits in the worm that were involved with the sense of touch. If you touch a worm on its nose, it will crawl backwards to try to escape. It turned out to be in an ion channel that responds to mechanical stimuli that’s important for the sense of touch. She found that when you knock out this gene, the mutants were defective in this escape response. The next step was to try to rescue the mutant phenotype in particular neurons, and that tells you in which neurons this gene has to function in order to have normal behaviour.
She started doing these rescue experiments, and there was one neuron that everybody knew was the was the most important neuron for this escape behaviour, because if you kill it with a laser, the worms don’t do the behaviour. However, when she did the rescue experiments, it didn’t rescue. On the other hand, when she tried to put the wild type gene back in other neurons, she did see rescue. This was weird because they weren’t supposed to be important for this escape behaviour. It was really very puzzling, and we didn’t really quite know how to understand this result.
It was only a few years later, when I had another student in the lab, that we identified that there was another circuit where these supposedly unimportant neurons were connected by something called gap junctions. It turned out that there was this mechanism of coincidence detection, where you had to activate multiple neurons that were connected by gap functions into this escape circuit, and that would give you this behaviour. The experiments where you killed those neurons with a laser didn’t give the same result as changing the activity of those neurons with this mutation. This was a case where something gave a weird result but actually led to an unexpected insight in how these circuits worked.
William was interviewed for the LMB Alumni Newsletter on 11th November 2024