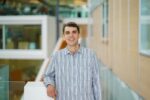
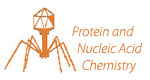
Starts as a Group Leader July 2025
A direct consequence of the universality of the genetic code is the possibility for genetic information to be transferred between evolutionarily distant species. Such horizontal transfer of genetic information is common in nature and has shaped evolution over billions of years. In the context of genetic engineering, however, this type of genetic spillover is highly concerning. Prevention of interference of artificial genetic information with natural biology is critical to allow biotechnological progress to be both safe and ambitious.
Furthermore, biotechnology will play a central role in addressing pressing challenges in food security, pharmaceutical development, sustainable fuel sources, and efficient carbon fixation. Thus, essential parts of the economy will increasingly rely on bioproduction facilities harboring tailor-made microbes. It is therefore critical that such facilities are extremely reliable. However, due to the universality of the genetic code, engineered organisms are just as susceptible to viral invasion as natural organisms. In fact, a single viral particle that finds its way into a bioproduction facility can force its operational shutdown.
Altering the genetic code of a cell provides an opportunity to render natural and synthetic genetic information incompatible. This breakthrough offers a means to protect the environment from genetically engineered organisms and, vice versa, engineered organisms critical for bioproduction from viral invasion. Through concerted efforts in genome recoding and translational engineering, it was possible to create the first organism with a synthetic genetic code. Since this organism “speaks a different language” than organisms found in nature, it is genetically isolated; it can neither give nor receive genetic information from the environment.
The lab is continuing to develop altered genetic codes to increase the safety of biotechnology and hopes to rewrite even the most complex biological systems in alternative synthetic genetic codes.
Altering fundamental cellular properties, such as the genetic code relies on the ability of defining every single base in the genome of a cell. Central to this effort is the ability to synthesize entire cellular genomes.
However, current genome synthesis methods are slow, narrow in scope, and limited in scale. To date, the genomes of only two bacteria have been successfully synthesized. The largest genome ever synthesized (E. coli strain Syn61) stands at 4 million letters. The genomes of higher organisms, such as mammals and plants, however, are on the scale of up to billions of letters.
The lab is developing methodologies to i) make the synthesis of model organism genomes (i.e. E. coli) more rapid, ii) enable the synthesis of the genomes of non-model bacteria to broaden the scope of genome synthesis, and iii) work towards the synthesis of genomes of eukaryotic organisms.
The ability to routinely synthesize the genomes of a diverse set of organisms will not only allow reprogramming of the genetic code but also facilitate genome modularization and minimization. Ultimately, genome synthesis will enable biological design at the organism scale with implications in bioproduction, human health, agriculture, and beyond.
Selected Papers
- Zürcher, J.F., Robertson, W.E., Kappes, T., Petris, G., Elliott, T.S., Salmond, G.P.C., Chin, J.W. (2022)
Refactored genetic codes enable bidirectional genetic isolation
Science 378: 516-523 - Zürcher, J.F., Kleefeldt, A.A., Funke, L.F.H., Birnbaum, J., Fredens, J., Grazioli, S., Liu, K.C., Spinck, M., Petris, G., Murat, P., Rehm, F.B.H., Sale, J.E., Chin, J.W. (2023)
Continuous synthesis of E. coli genome sections and Mb-scale human DNA assembly
Nature 619: 555-562